The buildings and construction sector accounted for 36% of final global energy use in 2018[1]. This staggering share of the world’s energy trickles down to a large share of Greenhouse Gas (GHG) emissions where buildings accounted for 39% of the world’s emissions in that same year. It is important to highlight that over a quarter of these emissions came from manufacturing essential products needed for building constructions such as steel, cement, and glass.
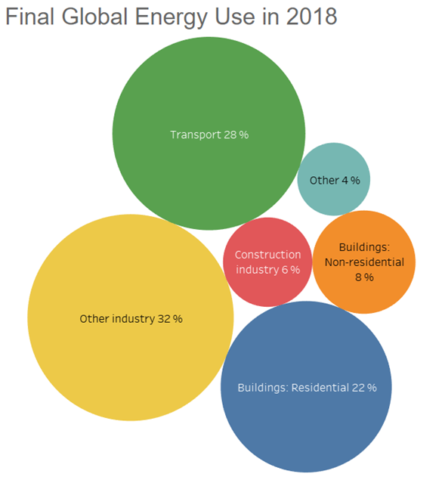
The buildings sector is very different from other economic sectors. The buildings sector is characterized with a slow pace of change, it is usually associated with relatively high capital costs which stems from high value assets tied to buildings such as land and utilities services. The construction of buildings is also an industry that can have high labor, operating, and capital costs in some geographical regions. The Building and Construction sectors are regulated by national and municipal codes, and these factors make the decision making process, that are tied to buildings’ capital projects or expenses, longer. The adoption curve of new and transformative technologies are less steep in buildings because of these sector characteristics. This is definitely a challenge for low carbon technology transformation for buildings, however, in this article we will focus on technologies that would enable the transformation of buildings to low carbon buildings.
“A quarter of global building emissions comes from activities associated with construction of buildings.”
Low Carbon Energy Technology for Buildings
Building energy consumption depends on the activities inside the building. Generally speaking, for any building, there is a base energy use associated with heating, cooling, and lighting. These are items that almost always exist in all buildings. Unfortunately, maintaining energy efficiency of these energy consumers is often overlooked, especially for older buildings. It is important to mention that lighting efficiency, as an example, has been making impressive strides in the past few years, and this is because of the relative ease of addressing lighting technology efficiency in buildings. Lighting is simply the low hanging fruit of energy conservation. However, when looking at building heating and cooling it becomes more difficult to further transform these energy consumers to low carbon technologies. In addition, waste energy in buildings is often overlooked, solid waste is often seen as an expense item, and waste energy utilization is usually not taken into account in building operations.
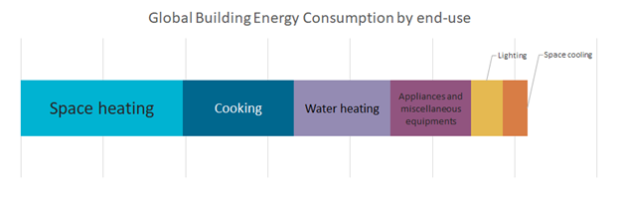
The current reality is that net-zero energy buildings have been touted as a pathway to better buildings, where the net-zero energy concept incorporates renewable energy generation on-site, or offsite, to address building energy consumption. Nonetheless, such concepts are far from mass adoption.
Embodied carbon[2] is another overlooked item when it comes to low carbon buildings. The transformation to low carbon faces astounding challenges to reduce carbon from upstream operations that are not visible to building users and operators, this carbon footprint is harder to quantify, measure, verify, and report. For example, building construction, and its associated supply chain of materials and manufacturing, contribute to a building’s carbon footprint if such operations have a carbon footprint (in 2021 they almost always do). This means that such a building would have a “day zero GHG footprint” even though the building doesn’t use any fossil fuels in its operation throughout its lifecycle. This presents a real challenge to building new zero-carbon buildings as well as transforming existing buildings to low carbon ones.
On the bright side, both new and existing buildings could benefit from low carbon technologies to achieve lower carbon operations. New low carbon building technologies will play a key role in the transformation for buildings to low carbon energy. Several technologies are prime candidates for this transformation, and we see this transformation happening in two waves:
The First Wave
Low carbon building technologies, which are easier to implement at scale in the next 5 to 10 years, will be technologies that achieve some level of maturity as well as proven reliability and cost competitiveness. For example, building electrification technologies will involve converting on-site GHG emitters to electricity, this reduces building on-site GHG emissions (Scope 1) and for electricity grids that utilize low carbon energy sources it reduces upstream emissions (Scope 2). Building heating electrification will involve using heat pumps, electric heating which will boost the energy efficiency of these heating demands and allow growth in such demand with minimal impact on GHG footprint. However, it will also involve significant increases in building electric load requirements which becomes a challenge to grid operators. The challenge to the electric grid becomes more difficult when full building electrification includes Electric Vehicle (EV) charging into the mix. EV charging challenge comes with an exciting opportunity for two way grid integration for EV batteries into the smart electricity grid of the future. Electrification technologies will enable more adoption of larger scale building battery energy storage which will increase building and grid resilience, and help buildings respond to unexpected events. The transition to low carbon energy buildings will also enable energy storage technologies at large to be more widely deployed, and the transition to heat pump heating will open the door for opportunities for larger scale energy storage technologies other than battarties (i.e. thermal energy storage, gravity energy storage, synthetic fuel).
Distributed shared energy systems for buildings will make most sense in densely populated areas, while off-grid renewables and low carbon energy systems will present better opportunities for less densely populated and rural areas. Solar photovoltaic (PV) and micro wind turbines will have to make significant strides in efficiency and cost for them to become feasible in buildings which are in densely populated areas, however they can play some role for less densely populated areas.
Buildings transition to lower carbon energy sources and operation can also enable a series of “ad-hoc” technologies that complement this transition. For example, advanced building automation systems that use state of the art algorithms to control and predict building operation can further enhance the efficiency of the newer low carbon systems. Adding the advanced building connectivity technology can also add a further advantage to newer low carbon energy buildings. This can help building users and operators understand and interact with buildings in a manner that further improves buildings transition to a low carbon future.
The last component of the first wave technologies for building transition to low carbon is the use of fuels that have lower carbon intensity. These fuels can help in the interim in lowering building emissions. Using biomass, biogas, and renewable natural gas can decrease carbon footprint for building heating while the transition from use of coal and oil to natural gas can help reduce the carbon footprint of buildings that heavily rely on these sources in their operations.
The Second Wave
The second wave of low carbon building technologies will be critical to reaching the finish line. This wave of technologies may play a significant role in the following 10 to 20 years (hopefully earlier). It is important to note that for the second wave of low carbon technologies to fully penetrate the building sector, it will involve other factors that are not technological in nature.
Renewable energy carriers could play a role where it makes the most sense. Traditionally, electricity has been the prominent carrier of renewable energy from wind and solar energy generation. However, other lower efficiency energy carriers like Ammonia and Hydrogen are being contemplated to bridge the gap where direct electricity transmission and battery energy storage fails to deliver the required efficiency, energy density, or the availability when renewable energy is needed. These carriers would need to deliver order of magnitude efficiency and cost improvements to deliver any value to the low carbon transition of buildings.

The industrial building sector’s hunger for higher temperature heat and large electric capacities make Small Modular Reactors (SMR) prime candidates as a lower carbon energy technology for industrial use and for harder to electrify buildings that have high temperature heat requirements. However, the SMR technology may face challenges such as slower regulatory process, unproven construction and financing economics, human talent constraints, as well as uncertainty in public perception when it comes to this specific new technology adoption. On the other hand, geothermal energy technology can satisfy the industrial building low carbon energy transition requirements and does not face the public perception risk, but indeed faces economic challenges as well as geographical constraints. These technologies are discussed in more detail here and here.
Finally, low carbon building technologies are on the horizon and being phased into buildings at a specific pace that is industry driven. However, the success of this transition will depend on both technological superiority as well as the suitability of these technologies where they are implemented. In the next article we will tackle technologies that pertain to the transportation sector.
If you learned something new or liked this content follow me here
For questions, showing support, and feedback contact me here
[1] “2019 Global Status Report for Buildings and Construction Sector ….” 11 Dec. 2019, https://www.unep.org/resources/publication/2019-global-status-report-buildings-and-construction-sector.
[2] “Embodied Carbon: What you Can Do Right Now – AIA California.” 5 Mar. 2020, https://aiacalifornia.org/embodied-carbon-definitions-and-facts/. Accessed 3 Apr. 2021.